The Power of Hybridization Explained: 15. Use Hybridization to Explain Why Carbon Can Only Form Four Bonds.
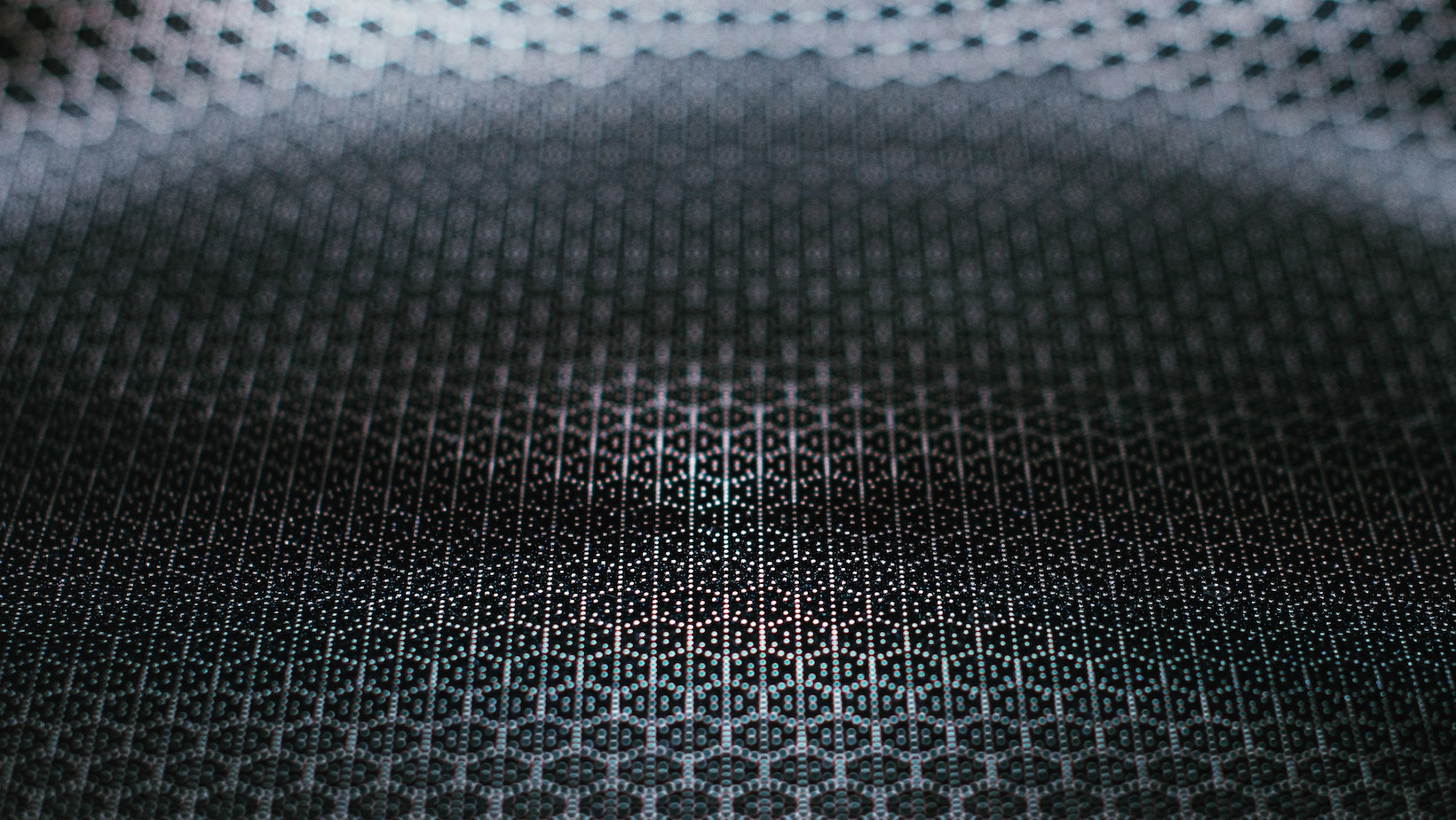
When it comes to understanding why carbon can only form four bonds, hybridization plays a crucial role. Hybridization refers to the mixing of atomic orbitals to form new hybrid orbitals that allow for bonding. In the case of carbon, its electronic configuration (1s^2 2s^2 2p^2) suggests that it has four valence electrons available for bonding.
15. Use Hybridization to Explain Why Carbon Can Only Form Four Bonds.
Carbon’s electron configuration includes two electrons in the 2s orbital and two in separate 2p orbitals. To maximize its bonding capacity, these orbitals undergo hybridization. Specifically, one of the 2s electrons and three of the 2p electrons combine to form four new sp3 hybrid orbitals. The resulting hybridized orbitals are arranged in a tetrahedral geometry around the carbon atom.
This tetrahedral arrangement allows each sp3 orbital to overlap with an orbital from another atom, forming covalent bonds. Since there are four sp3 hybrid orbitals available, carbon can bond with up to four other atoms or groups of atoms, resulting in its ability to form four bonds.
Understanding Hybridization
When it comes to understanding why carbon can only form four bonds, hybridization plays a crucial role. Hybridization is the mixing of atomic orbitals to form new hybrid orbitals that are better suited for bonding. In the case of carbon, its electron configuration of 1s² 2s² 2p² allows it to form four valence bonds.
Carbon’s ability to form multiple bonds is due to its unique electronic structure and its capacity for hybridization. The ground state configuration of carbon consists of two electrons in the s orbital (2s) and two in the p orbital (2p). However, in order to have access to four unpaired electrons for bonding, carbon undergoes sp³ hybridization.
During sp³ hybridization, one electron from the s orbital and three electrons from the p orbitals are combined to create four equivalent sp³ hybrid orbitals. These new orbitals have a tetrahedral arrangement around the carbon atom, with each orbital pointing towards one corner of a tetrahedron.
The resulting sp³ hybridized orbitals allow carbon atoms to participate in covalent bonding by overlapping with other atomic orbitals. This overlap leads to the formation of sigma bonds between carbon and other atoms such as hydrogen or oxygen. Additionally, these sp³ hybrids provide an ideal arrangement for forming single bonds with other atoms.
It’s important to note that while carbon predominantly forms covalent bonds through sp³ hybridization, it can also exhibit other types of hybridizations such as sp² or even pure p character depending on the specific molecular environment.
The Basics of Carbon Bonding
When it comes to understanding why carbon can only form four bonds, the concept of hybridization plays a crucial role. Hybridization refers to the process in which atomic orbitals combine to form new hybrid orbitals that have different shapes and energies. In the case of carbon, hybridization allows it to achieve a stable state by forming four covalent bonds.
Carbon has an atomic number of 6, meaning it has six electrons. In its ground state, carbon’s electron configuration is 1s^2 2s^2 2p^2. The two electrons in the 1s orbital are closest to the nucleus, while the remaining four electrons are distributed among the 2s and 2p orbitals.
To maximize stability and minimize energy, carbon undergoes sp3 hybridization. This involves promoting one electron from the 2s orbital to an empty 2p orbital, resulting in four half-filled sp3 hybrid orbitals. These new orbitals have a tetrahedral arrangement around the carbon atom.
Each sp3 hybrid orbital can overlap with another atom’s valence orbital (usually another carbon or a different element) to form a sigma bond. This type of bond is characterized by head-on overlap between atomic orbitals along their axis.
Thanks to this unique arrangement and bonding capability afforded by hybridization, carbon can create strong covalent bonds with up to four other atoms simultaneously. This versatility is what makes carbon such an essential element for life on Earth as it forms the backbone of organic molecules like carbohydrates, proteins, lipids, and nucleic acids.
In conclusion, while hybridization theory serves as a useful tool to understand carbon’s ability to form four bonds, it is crucial to acknowledge its limitations. By recognizing these limitations and exploring alternative theories, we can continue to deepen our understanding of chemical bonding and the intricacies of molecular structures.